Comparative Study of the Effect of Four (4) ISCO Oxidants on PCE Oxidation and Aerobic Microbial Act
Learn how Cool-Ox® oxidized 96% and 95% of PCE & EB respectively while promoting the growth of native microorganisms.
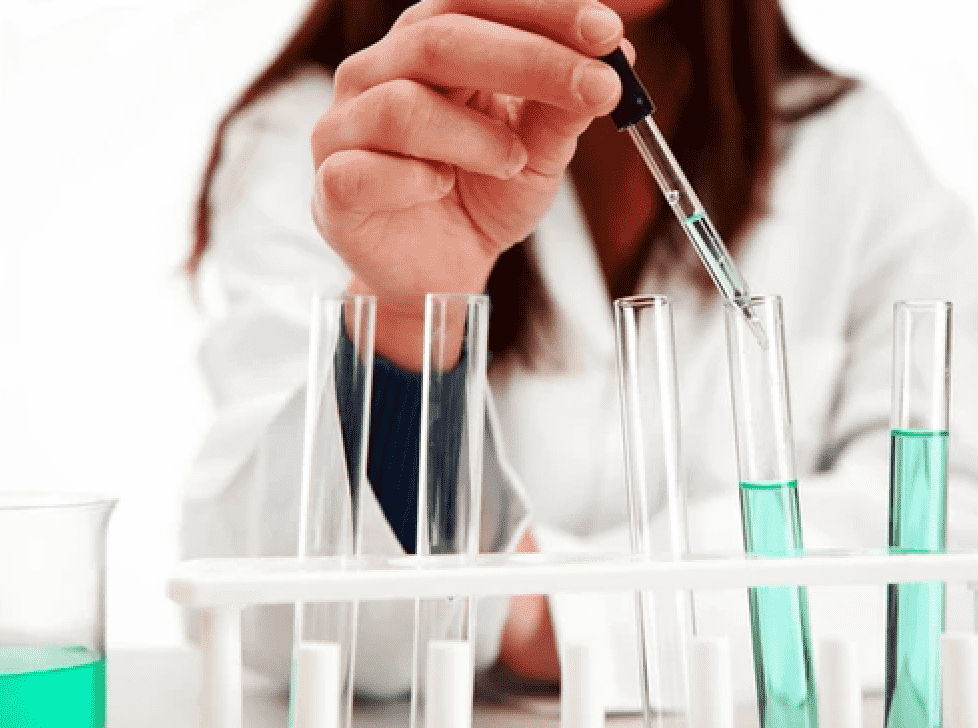
Updated: Nov 30, 2021
Joseph Bou-Nasr and Dan Cassidy (dcassidy@ggl.ulaval.ca) (Laval Univ., Canada)
Duane Hampton (Western Michigan University, USA)
Abstract
A comparative study was done to test the effectiveness of four chemical oxidants to treat soil slurries artificially contaminated with tetrachloroethylene (PCE) and ethylbenzene (EB). Four oxidants were tested; (1) potassium permanganate, (2) a modified Fenton system using liquid hydrogen peroxide (H2O2 or HP) activated with chelated Fe(III), (3) a commercially available, calcium peroxide (CaO2)-based modified Fenton (MF) product (Cool-Ox®), and (4) sodium persulfate activated Fe(II) with a chelator.
The CaO2-based Fenton (MF) product (Cool-Ox®) supplied by DeepEarth Technologies, Inc. resulted in the greatest destruction of PCE and EB, 96% and 95%, respectively. Permanganate oxidized 89% of the PCE, but only 10% of the EB. Liquid HP-based Fenton reactions showed the greatest initial rates of reaction, but only oxidized 81% and 74%, respectively. Persulfate only oxidized 31% of the PCE and 15% of the EB. In addition to measuring contaminant removal, the effect of the oxidants on counts of culturable indigenous heterotrophic microorganisms in the soil was investigated. The CaO2-based product (Cool-Ox®) increased microbial counts nearly an order of magnitude, while the liquid HP system reduced counts by two (2) orders of magnitude. Permanganate and persulfate oxidation did not affect microbial counts significantly.
Introduction
In situ chemical oxidation (ISCO) is a powerful tool for the destruction of many environmental contaminants. The most common oxidants used in ISCO applications are modified Fenton chemistry, permanganate, and persulfate (Watts and Teel, 2006). The permanganate ion (MnO4-) oxidizes contaminants directly, and can be added as the potassium or sodium salt. Modified Fenton treatment uses hydrogen peroxide (H2O2, or HP) to produce hydroxyl radicals at a quasi-neutral pH (in contrast with conventional Fenton chemistry which uses a pH below 3). Iron is required to “activate” the HP, producing the hydroxyl radicals necessary for contaminant oxidation. There are variations in how H2O2 and iron are provided to soils in Fenton systems. Iron can be added as salts of Fe2+ or Fe3+ (Watts and Dilly, 1996), or native iron-containing minerals (e.g., goethite, ferrihydrite, magnetite) can be used (Kong et al., 1998; Yeh et al., 2003). Organic chelating agents can be added to modified Fenton systems to increase rates of hydroxyl radical production by enhancing the presence of Fe3+ in the aqueous phase (Sun and Pignatello, 1992; Nam et al., 2003), though this increases costs.
An alternative to liquid HP is adding calcium peroxide (CaO2), which releases H2O2 as it dissolves in water (Schumb et al., 1955, Arienzo, 2000, Bogan et al., 2003). Persulfate (peroxydisulfate) is relatively new to ISCO. The persulfate ion (S2O82-) itself is not a strong oxidant, but when properly activated by iron, heat, and/or extreme pH values, persulfate decomposes to form the sulfate radical, which is one of the most powerful oxidizing agents known (Watts and Teel, 2006). Activating persulfate to form sulfate radicals has limited its application in ISCO.
In these studies, a soil was artificially contaminated with tetrachloroethylene (PCE) and ethylbenzene (EB) and was then treated in slurry reactors with the following oxidants; (1) potassium permanganate (KMnO4), (2) a modified Fenton system using liquid HP, (3) a CaO2–based modified Fenton oxidant (Cool-Ox®), and (4) sodium persulfate (Na2S2O8). The first objective of this study was to compare the effectiveness of four oxidants in treating both PCE and EB. The EB was added because most laboratory studies focusing on PCE or trichloroethylene (TCE) use these test contaminants only, whereas the majority of sites contaminated from drycleaning and degreasing activities also have petroleum contamination.
The second objective of this work was to investigate the response of native soil microorganisms to the four chemical oxidants tested. This becomes important when considering that readily biodegradable compounds (e.g., oxalate, formate, and other low molecular weight fatty acids) are known to form during the oxidation of most organic contaminants with both permanganate and modified Fenton chemistry. Moreover, the H2O2 used in Fenton reactions also releases molecular oxygen, which can be used for aerobic biodegradation if the microorganisms are not killed or inactivated. It is critical to know whether excessive death and/or inactivation of microorganisms occurs during chemical oxidation, which affects their capacity to degrade daughter products and any remaining contaminants that might be detrimental to the long-term health of a soil. Several recent studies have indicated that chemical oxidation of contaminants in soils can take place simultaneously with aerobic heterotrophic metabolism, or at least without inactivating soil microorganisms for long periods of time (Ndjou’ou et al., 2006; Ndjou’ou and Cassidy, 2006).
Materials & Methods
An uncontaminated soil was sampled and then sieved (0.2 mm) to remove all but the fine sand, silt and clay fractions. The characteristics of the sieved soil used in the studies are listed in Table 1. A slurry (40% solids) was prepared with the sieved soil, which was buffered at the natural soil pH (6.5) with a phosphate buffer. The slurry was then artificially contaminated and split into four different reactors for treatment. The slurry reactors were four (4) liter glass vessels with fitted covers housing a mechanical mixer, a pH probe, and a Supelco ORBO tube to collect stripped PCE and EB. The slurry was mixed but not sparged with air.
Potassium permanganate was added at a dose of 10 g MnO4-/kg soil. The liquid HP-based modified Fenton oxidation system consisted of adding H2O2 at a dose of 10 g H2O2/kg soil. The HP was activated with Fe(III) using ethylenediaminetetraacetic acid (EDTA) as a chelator at a molar ratio of H2O2:Fe(III):EDTA of 20:1:1. The CaO2-based treatment consisted of adding 10 g of the oxidizing powder/kg soil. The powder was (Cool-Ox®) obtained from DeepEarth Technologies, Inc. (Alsip, IL, USA). Persulfate was added at a dose of 10 g S2O8 2-/kg soil, and was activated with chelated Fe(II) with EDTA at a molar ratio of S2O8 2-:Fe(II):EDTA of 20:1:1.
Chloride ion (Cl-) concentrations were measured in duplicate slurry filtrate samples with a Thermo Orion chloride ion specific electrode and a 720APlus meter. PCE and EB were analyzed in duplicate hexane extracts using a Hewlett-Packard 6890 gas chromatograph (GC) with electron capture detection. Heterotrophic plate counts were done on trypticase soy broth agar (TSBA).

Results & Discussion
Chemical Oxidation of PCE and EB
The first objective of this study was to compare the effectiveness of four oxidants in treating PCE and EB. Figures 1 and 2 illustrate the variation with time of concentrations of PCE and EB, respectively, over the 2,880 minute (48 hour) study period. Table 2 lists the PCE oxidized, Cl- yield per mole of PCE oxidized, and EB oxidized after treatment with the different oxidants tested.
Stripping of PCE and EB (data not shown) was less than 6% of the total loss of PCE and less than 4% of the total loss of EB in all the reactors. The percentages of PCE and EB oxidized were calculated from the difference between the total removal and the mass stripped and recovered in the ORBO tubes. The Cl- measurements in slurry filtrate allowed the molar ratio of Cl- released per PCE oxidized. The measured release of between 3.6 and 3.9 moles Cl- per mole PCE oxidized indicates that the PCE was in fact chemically oxidized and that mineralization of the PCE occurred. The pH values are not reported but, remained at approximately 6.5 in all the reactors throughout the studies.
The control reactor did not show significant PCE or EB removal (Figures 1 and 2). The CaO2-based Fenton product (Cool-Ox®) showed the greatest destruction of PCE (96%) and EB (95%) (Table 2), though rates of oxidation were considerably less than initial rates for the liquid HP-based Fenton and permanganate systems. The liquid HP Fenton system showed the greatest initial oxidation rates of PCE and EB, but the oxidation reactions stopped almost completely after 60 to 100 minutes. In a study comparing DNAPL oxidation with permanganate and liquid HP-modified Fenton systems, Crimi and Siegrist (2005) reported that HP is less stable than permanganate, but more efficient (i.e., less oxidant is required to achieve the same contaminant removal). The results in Figure 1 seem to be consistent with this. Liquid HP showed higher initial rates of PCE oxidation but, stopped short of complete removal because of its instability relative to permanganate and the CaO2-based (Cool-Ox®) product. Figure 1 shows that the CaO2-based product (Cool-Ox®) provided more stability than even permanganate. Oxidant stability is a major factor in the
success of ISCO, because unstable oxidants cannot be transported effectively away
from the injection points to the interior of the aquifer.
When comparing the two modified Fenton systems, the instability of H2O2 also explains the better performance of the CaO2-based (Cool-Ox®) treatment compared with liquid HP. These results clearly show that the CaO2-based product (Cool-Ox®) sustained chemical oxidation over a significantly longer period of time than the liquid HP treatment, and achieved greater overall contaminant removal. The reason for this is that the H2O2 required for the Fenton reactions is released slowly from CaO2 as it dissolves in water, instead of being available all at once as with liquid HP. Consistent with this is the observation that foaming was much more intense, though short-lived (1-2 hours), in the reactor with liquid HP than in the one with the CaO2-based (Cool-Ox®) product. In similar studies on a petroleumcontaminated soil, Ndjou’ou and Cassidy (2006) also observed that a CaO2-based Fenton (Cool-Ox®) system sustained chemical oxidation reactions longer than a liquid HP and resulted in significantly greater hydrocarbon removal.
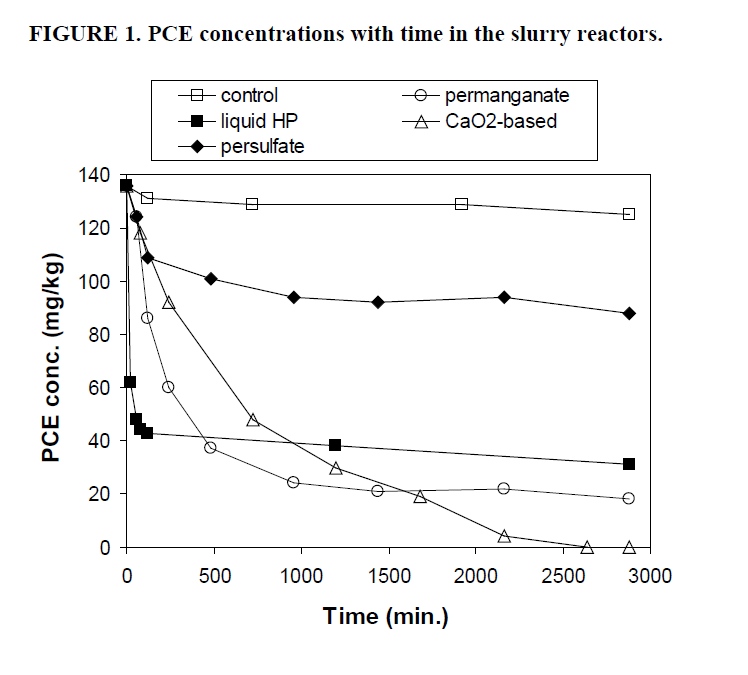
Potassium permanganate oxidized 89% of the PCE, performing nearly as well as the CaO2-based (Cool-Ox®) product. However, only 10% of the EB was oxidized by permanganate. These results indicate that permanganate is not an appropriate choice of oxidant for sites having mixed contamination with chlorinated solvents and hydrocarbons. Permanganate is often the oxidant of choice for ISCO systems treating chlorinated ethylenes, because the permanganate ion has an affinity for oxidizing the carbon-carbon double bond in these compounds (Schnarr et al., 1998). Permanganate is also effective at oxidizing some polycyclic aromatic hydrocarbons (Gates-Anderson, et al., 2001). However, permanganate is ineffective at oxidizing aromatic compounds (e.g., EB, benzene, xylenes, toluene) (Waldemer and Tratnyek, 2006).

Persulfate did not result in significant oxidation of either contaminant. Persulfate oxidized only 31% of the PCE and 15% of the EB. It is not known why persulfate did not perform well in these studies, but the poor results suggest that the persulfate was not activated to form sulfate radicals. Liang et el. (2004) used a similar chelated Fe(II) system to activate persulfate for the oxidation of TCE, and achieved nearly complete TCE removal. The Fe(II) is oxidized during the chemical reactions to produce Fe(III), which also occurs in Fenton reactions. The Fe(III) requires a chelator to stay in the aqueous phase because of its low solubility at quasi-neutral pH. The ratio of S2O82-:Fe(II):citric acid (20:1:1) used by Liang et el. (2004) was identical to the ratio used in this study, except the citric acid was replaced with EDTA. Citric acid is not considered to be nearly as effective at chelating Fe as EDTA (used in this study). These results underscore the difficulty in properly activating persulfate to
ensure that sulfate radicals are formed and indicate that more research is needed to develop persulfate oxidation as a reliable tool for ISCO systems.
Effect of Oxidants on Native Soil Microorganisms
The second objective of this work was to investigate the response of native soil microorganisms to the four chemical oxidants tested. Table 2 lists the heterotrophic plate counts after treatment for 48 hours with the four chemical oxidants. The counts for the control reactor were 7.51 log units/g soil (Table 2), which is quite similar to the value of 7.69 log units/g measured in the sieved soil (Table 1). The CaO2-based (Cool-Ox®) product increased microbial counts nearly an order of magnitude from these background levels to 8.31 log units/g, while the liquid HP system reduced counts by approximately 2 orders of magnitude to 5.42 log units/g. Because PCE is not readily biodegradable aerobically, the increase in counts observed for the CaO2-based (Cool-Ox®) product was likely due to growth on products from the partial oxidation of native organic material and/or from PCE. Permanganate and persulfate oxidation did not affect counts significantly. The results obtained for the two Fenton oxidation systems are consistent with those reported by Ndjou’ou and Cassidy (2006). They found that the CaO2-based (Cool-Ox®) product slightly increased the microbial counts while treating a soil contaminated with petroleum hydrocarbons, whereas a liquid HP system reduced microbial counts.

The microbial counts in Table 2 are significant because they show that chemical oxidation did not kill or inactivate most microorganisms in the soil, even for the liquid HP system. Though some microorganisms are certainly killed or inactivated by chemical oxidants, these results indicate that microbial populations in ISCO are not necessarily reduced considerably. Arienzo (2000) found that phospholipid levels (a surrogate measure of viable biomass) were unaffected or increased after treating a trinitrotoluene-contaminated soil with a CaO2-based modified Fenton oxidant. Ndjou’ou and Cassidy (2006) showed that modified Fenton (Cool-Ox®) oxidation of hydrocarbons in soil did not significantly reduce plate counts of native hydrocarbon-degraders.
In studies using modified Fenton oxidation of tetrachloroethylene (PCE) with liquid HP, Ndjou’ou et al. (2006) showed that doses 20 times that required to achieve 95% PCE removal only slightly reduced plate counts of indigenous microorganisms, and only delayed the onset of aerobic metabolic activity a few hours. Even conventional Fenton treatment (pH=2) in a slurry reactor only reduced bacterial counts from 108 to 104 per mL (US EPA, 1997). In contrast, Schrader and Hess (2004) reported a reduction in counts of soil bacteria of nearly 106 log units/g soil after modified Fenton oxidation in soil slurries.
The microbial count results also suggest that chemical oxidation can take place simultaneously with biological oxidation. Biological processes have traditionally been considered incompatible with chemical oxidation because of excessive death and/or inactivation of the native microorganisms. However, results from recent studies have demonstrated that modified Fenton reactions (Cool-Ox®) can co-exist with aerobic microbial activity. Ndjou’ou et al., (2006) demonstrated that aerobic heterotrophic activity took place simultaneously with chemical oxidation of PCE using a liquid HP-based modified Fenton system. Oxygen released from the H2O2 during chemical oxidation was used simultaneously by soil microorganisms for aerobic metabolic activity. Büyüksönmez et al. (1999) showed that addition of X. flavus to a modified Fenton system oxidizing PCE resulted in 10% greater mineralization than stand-alone reactions. Howsawkeng et al. (2001) also found evidence for simultaneous chemical oxidation of PCE and aerobic assimilation of oxalate (OA) by X. flavus in the presence of modified Fenton reactions. The results from this study, and from several others suggest that in the future ISCO systems can be designed to optimize both chemical and biological degradation processes.
Conclusions
Of the four chemical oxidants tested to treat PCE and EB in soil slurries, the CaO2-based modified Fenton (Cool-Ox®) product performed the best, oxidizing 96% and 95% of PCE and EB, respectively. In addition, this oxidant was the most stable, and increased counts of native soil microorganisms. A liquid HP-based modified Fenton system showed high initial oxidation rates, but stopped suddenly after oxidizing 81% of PCE and 74% of EB. Liquid HP also reduced microbial counts nearly two orders of magnitude. Permanganate oxidized 89% of the PCE, but only 10% of the EB. Persulfate was the least effective, oxidizing only 31% of PCE and 15% of EB.
Below is a video animation of Cool-Ox® reacting with PCE
Acknowledgements
We thank the National Science and Engineering Research Council of Canada for funded this research.
References
Arienzo, M., 2000. “Degradation of 2,4,6-trinitrotoluene in water and soil slurry
utilizing a calcium peroxide compound.” Chemosphere. 40: 331-337.
Bogan, B.W., Trbovic, V., and J.R. Paterek. 2003. “Inclusion of Vegetable Oils in
Fenton’s Chemistry for Remediation of PAH-Contaminated Soils.” Chemosphere.
50: 12-21.
Büyüksönmez, F., Hess, T.F., Crawford, R.L., Paszczynski, A., and R.J. Watts. 1999.
“Optimization of Simultaneous Chemical and Biological Mineralization of
Perchloroethylene.” Appl. Environ. Microbiol. 65: 2784-2788.
Crimi, M.L., and R.L. Siegrist. 2005. “Factors Affecting Effectiveness and Efficiency
of DNAPL Destruction Using Potassium Permanganate and Catalyzed Hydrogen
Peroxide.” J. Environ. Engineering (ASCE), 131(12): 1724-1732.
Gates-Anderson, D.D., Siegrist, R.L., and S.R. Cline. 2001. “Comparison of
Potassium Permanganate and Hydrogen Peroxide as Chemical Oxidants for
Organically Contaminated Soils.” J. Environ. Engin. (ASCE). 127(4): 337-347.
Howsawkeng, J., Watts, R.J., Washington, D.L., Teel, A.L., Hess, T.F., and R.L.
Crawford. 2001. “Evidence for Simultaneous Abiotic-Biotic Oxidations in a
Microbial-Fenton’s System.” Environ. Sci. Technol. 35: 2961-2966.
Kong, S.-H., Watts, R.J., and J.-H. Choi. 1998. “Treatment of Petroleum-
Contaminated Soils Using Iron Mineral Catalyzed Hydrogen Peroxide.”
Chemosphere. 37: 1473-1482.
Liang C., Bruell, C.J., Marley, M.C., and K.L. Sperry. 2004. “Persulfate Oxidation
for In Situ Remediation of TCE. II. Activated by Chelated Ferrous Iron.”
Chemosphere, 55:1225-1233.
Nam, K., Rodriguez, W., and J.J. Kukor. 2001. “Enhanced Degradation of Polycyclic
Aromatic Hydrocarbons by Biodegradation Combined with a Modified Fenton
Reaction.” Chemosphere. 45: 11-20.
Ndjou’ou A-C, Bou-Nasr, J, and D.P. Cassidy. (in press). “The Effect of Fenton
Reagent Dose on Co-Existing Chemical and Microbial Oxidation in Soil.”
Environ. Science & Technol.
Ndjou’ou A-C, and D.P. Cassidy. (in press). “Surfactant Production Accompanying
the Modified Fenton Oxidation of Hydrocarbons in Soil.” Chemosphere.
Schnarr, M., Traux, C., Faruhar, G., Hood, E., Gonully, T., and B. Stickney. 1998.
“Laboratory and Controlled Field Experimentation Using Potassium
Permanganate to Remediate Trichloroethylene and Perchloroethylene DNAPLs in
Porous Media.” J. Contam. Hydrol. 29(3): 205-224.
Schrader, P.S. and T.F. Hess. 2004. “Coupled Abiotic-Biotic Mineralization of 2,4,6-
Trinitrotoluene (TNT) in Soil Slurry.” J Environmental Quality. 33: 1202-1209.
Schumb, W.E., Stratterfield, C.N., and R.L. Wentworth. 1955. Hydrogen Peroxide.
Van Nostrand Reinhold, New York.
Sun, Y., and J.J. Pignatello. 1992. “Chemical Treatment of Pesticide Wastes:
Evaluation of Fe(III) Chelates for Catalytic Hydrogen Peroxide Oxidation of 2,4-
D at Circumneutral pH.” J. Agric. Food Chem. 40: 332-337.
US Environmental Protection Agency (1997). Innovative Methods for Bioslurry
Treatment. SITE-Emerging Technology Summary. EPA/540/SR-96/505.
Waldemer, R.H., and P.G. Tratnyek. 2006. “Kinetics of Contaminant Degradation by
Permanganate.” Environ. Sci. Technol. 40: 1055-1061.
Watts, R. J., and A.L. Teel. 2006. “Treatment of Contaminated Soils and
Groundwater Using ISCO.” Practice Periodical of Haz., Toxic, Radioactive
Waste Management. 10(1): 2-9.
Watts, R.J., and S.E Dilly. 1996. “Evaluation of Iron Catalysts for the Fenton-Like
Remediation of Diesel-Contaminated Soils.” J. Haz Mat. 51: 209-224.
Yeh, C.K.-J., Wu, H.-M., and T.-C. Chen. 2003. “Chemical Oxidation of Chlorinated
Non-Aqueous Phase Liquid by Hydrogen Peroxide in Natural Sand Systems.” J
Haz Mat B96: 29-51.